Published on 2024/10/24 Research powered by Mightex’s Polygon1000
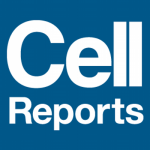
Introduction
Neuronal membranes consist of a phospholipid bilayer that separates the cytoplasmic and extracellular electrolytic media, acting as a capacitor dictating fundamental characteristics of neuronal activity, such as action potential axonal propagation speed and firing frequency 1,2. Capacitance is affected by the distance between intra- and extracellular media and the membrane surface area 3,4. It is widely accepted that membrane capacitance increases during neuronal development and becomes stable upon maturation due to myelination and perineuronal networks provide insulation, encapsulation and stability of membrane properties 5,6.
Findings and Conclusions
Contrary to widely accepted beliefs, Serevin and colleagues found circadian oscillations in membrane capacitance of 60 – 100% for both mature excitatory pyramidal neurons of layer 2/3 of the visual cortex and hippocampal dentate gyrus granule cells (Figure 1). The same finding was not shown in mature parvalbumin positive inhibitory neurons of the visual cortex (Figure 1).
Figure 1. Changes in membrane capacitance over zeitgeber time for pyramidal cells of the visual cortex, granule cells of the hippocampal dentate gyrus layer 2/3 and PV+ cells of the visual cortex. Adapted from Severin et al., (2024).
Using current-clamp whole cell electrophysiology, the authors demonstrated significant decreases in membrane capacitance from the end of the dark phase (Zeitgeber Time 0; ZT0) to the middle of the light phase (ZT6) to the end of the light phase (ZT12) for both visual cortex layer 2/3 pyramidal cells and hippocampal dentate gyrus granule cells.
While prior literature suggests that small but significant fluctuations across zeitgeber time in membrane capacitance for inhibitory neurons of the cortex may stem from daily changes in perineuronal net (PNN) formation, the authors did not see any significant circadian fluctuations in PV+ cells of the visual cortex in their recordings (Figure 1). Further, when PNNs were ablated, the authors found no difference in the neuronal capacitance of PV+ neurons across zeitgeber time, suggesting the influence of PNN on circadian membrane capacitance to be insignificant.
Membrane capacitance impacts how neurons integrate excitatory dendritic inputs. The authors used targeted optogenetics using the Mightex Polygon to explore the functional impact of circadian membrane capacitance oscillation on input integration.
In ChR2 expressing pyramidal cells, the authors applied sequential subthreshold 470nm stimulation targeted above and below the patched soma to mimic apical and basal excitatory inputs respectively. To interrogate the functional impact of capacitance changes, the time between the paired optical pulses was varied between 5 – 60ms (inter-pulse interval; IPI). For ZT0 cells, longer IPI was able to induce spiking, compared to ZT12 cells (Figure 2). A simplified computational model of these data confirmed that increase in membrane capacitance alone is sufficient to increase the temporal integration window of inputs to pyramidal cells.
Figure 2. Optogenetic interrogation of excitatory input integration for ZT0 and ZT12 cells. (A) Schematic of targeted optogenetics conducted using the Mightex Polygon, (B) optogenetic-induced action potentials with varying IPIs for ZT0 and ZT12 cells, (C) summary graph of probably of excitation for ZT0 and ZT 12 cells with varying IPIs. Adapted from Severin et al. (2024).
Use of Mightex Polygon
Severin and colleagues used the Mightex Polygon1000 integrated into a Nikon Eclipse E600FN microscope to deliver pulses of 3ms of 65 x 65 um2 covering selected apical and basal dendrites every 15s with the time between the apical and basal stimulation varying by a range of IPIs. Subthreshold intensity 470nm stimulation was used and CNQX, APV, and Gabazine were added to the ACSF to prevent off-target effects.
References
1. Hodgkin, A.L., and Huxley, A.F. (1952). A quantitative description of membrane current and its application to conduction and excitation in nerve. J. Physiol. 117, 500–544.
2. Golowasch, J., Nadim, F. (2014). Capacitance, Membrane. In: Jaeger, D., Jung, R. (eds) Encyclopedia of Computational Neuroscience. Springer, New York, NY. https://doi.org/10.1007/978-1-4614-7320-6_32-1
3. Baufreton, J., Atherton, J.F., Surmeier, D.J., and Bevan, M.D. (2005). Enhancement of excitatory synaptic integration by GABAergic inhibition in the subthalamic nucleus. J. Neurosci. 25, 8505–8517.
4. Martin, A.R. (1976). The effect of membrane capacitance on non-linear summation of synaptic potentials. J. Theor. Biol. 59, 179–187.
5. Castelfranco, A.M., and Hartline, D.K. (2015). The evolution of vertebrate and invertebrate myelin: a theoretical computational study. J. Comput. Neurosci. 38, 521–538.
6. Tewari, B.P., Chaunsali, L., Campbell, S.L., Patel, D.C., Goode, A.E., and Sontheimer, H. (2018). Perineuronal nets decrease membrane capacitance of peritumoral fast spiking interneurons in a model of epilepsy. Nat. Commun. 9, 4724.
To read the full publication, please click here.